Trans2DTMD Investigates Electronic Transport in Transition Metal Dichalcogenides
Lucian Covaci of the University of Antwerp details the characteristics and applications of transition metal dichalcogenides and the European project Trans2DTMD.
Graphene is at the core of the largest European research initiative to date, The Graphene Flagship, but within this megaproject there are also studies of other two-dimensional materials, such as transition metal dichalcogenides (TMD). The interesting properties of TMDs can be applied in electronics, spintronics and a third field: valleytronics, as the physicist Dr Lucian Covaci of the University of Antwerp explains in this interview.
At the last Graphene Week conference held in Athens in September 2017, more than 500 international experts in graphene, a carbon-based sheet material with enormous potential, gathered. The congress was also attended by researcher Dr Lucian Covaci as the representative of the Condensed Matter Theory group led by Professor François Peeters at the University of Antwerp, in Belgium.
Within the large European initiative Graphene Flagship, this group coordinates a project which does not focus on graphene but on other two-dimensional materials of growing interest: transition metal dichalcogenides (TMDs). Dr Covaci details the characteristics and applications of these novel materials.
What exactly are the TMDs?
They are a family of two-dimensional semiconductor materials with the formula MX2, where M represents a transition metal – an element in the central part of the periodic table, such as molybdenum (Mo) or tungsten (W) – and X represents a chalcogen – they are located in the column containing oxygen, such as sulphur (S), selenium (S) or tellurium (Te). Inserting a layer of atoms type M between two of type X we obtain the very thin yet stable monolayer of TMD. TMDs have been known for half a century in their three-dimensional version, but the graphene research of the last decade has also triggered interest in these two-dimensional materials and their properties.
What are the advantages of TMDs with regard to graphene?
There are many benefits of TMDs, and other two-dimensional materials like silicene, phosphorene or germanene, when compared to graphene, especially in electronics applications like field effect transistors. Within what is called bandgap energy, graphene is a zero-gap semiconductor, i.e. a semi-metal, and it can only be used as a contact or gate. One requires a tunable bandgap in order to develop a transistor, and most TMDs are semiconductors with sizable bandgaps.
Are there any other differences?
Another shortcoming of graphene is the fact that spin-orbit interactions are very weak, and therefore a lot of interesting physics related to spintronics is missing. This is not the case in most of the other two-dimensional materials, where the spin-orbit coupling is large, resulting in spin-split bands and the possibility of manipulating the spins of the electrons through electrical means. Nowadays, there is a strong push towards combining TMDs with graphene, inducing by proximity desired properties, like spin-orbit coupling.
And what is the European project you are working on now?
It is called Trans2DTMD, a three-year project that began in 2016. This is a theoretical investigation of electronic transport in two-dimensional and 'functionalised' TMDs, a term that refers to the fact that they are not pure, but have some element which changes their properties. The most interesting thing is that you can dope these materials by adding impurities (like introducing or varying some atom types) or changing its topology (at edges of nanoribbons and grain boundaries) to induce, for example, a metallic phase or magnetism.
And what are the main aims of Trans2DTMD?
On one hand, we develop novel numerical methods for electronic transport calculations in two-dimensional materials. We use both commercial numerical codes and proprietary tools developed by us to investigate the structural, optical and electronic characteristics of TMDs. On the other hand, we study the effects of defects and strains on the properties of metallic TMDs, as well as predicting novel two-dimensional materials with interesting properties.
What properties and applications do these TMDs have?
Their topological properties are of utmost importance in electronic applications, for example field effect transistors, photovoltaic devices and bio-sensors. TMDs are also relevant in spintronics, an emerging technology that exploits both the charge and spin of electrons, as well as in an even more novel one: valleytronics, where energy ‘valleys’ in the conduction band can trap and channel the electrons and act as a new degree of freedom.
What progress have you made so far?
To study these materials we are developing software based on the Pybinding code created at the University of Antwerp, which will be an open source available to the entire scientific community. We have so far implemented efficient codes that can take advantage of state-of-the-art computational resources: computer clusters and video cards. This will allow for the investigation of generic systems of disorder effects on the electrical and optical conductivity of two-dimensional materials.
What is this software for?
It will allow the simulation of very large systems (with billions of atoms) in order to capture effects that can then be observed in experimental samples. This is important since many of the theoretical tools fail to accurately study adatoms (individual atoms attached to a crystal) and defects that disperse the electricity. With this software, it will be possible to realise accurate spintronic and valleytronics simulations.
How many groups are involved in this project?
The Trans2DTMD project is coordinated by the Condensed Matter Theory group led by Professor François Peeters at the University of Antwerp, who have extensive experience in computational and theoretical tools to study TMDs. The second member of the consortium is the Theoretical Chemistry group led by Professor Thomas Heine at the University of Leipzig (Germany). Coordination with Graphene Flagship, and aspects related to graphene, is done through the Technical University of Dresden (Germany). In addition, as associated partners, the University of Twente (Netherlands) specialises in spintronics; and the University of the Basque Country provides calculations to analyze electron-electron interactions, an important aspect in two-dimensional materials.
Have Trans2DTMD members published any papers?
Together with other Graphene Flagship partners, the Leipzig University group has published a paper about optical quality improvements in molybdenum diselenide (MoSe2) in Nano Letters. They have also presented in 2D Materials calculations on potential and exotic properties of tantalum selenide (Ta2Se3), showing two Dirac points, rather than one as is the case of graphene. Within the effort to describe transport properties of two-dimensional materials, the University of Antwerp group has also submitted a study on the conductivity of twisted bilayer graphene for a wide range of angles of torsion and electric fields.
Defect Healing and Charge Transfer-Mediated Valley Polarization in MoS2/MoSe2/MoS2 Trilayer van der Waals Heterostructures. Alessandro Surrente, Dumitru Dumcenco, Zhuo Yang, Agnieszka Kuc, Yu Jing, Thomas Heine, Yen-Cheng Kung, Duncan K. Maude, Andras Kis, and Paulina Plochocka. Nano Lett., 2017, 17 (7), pp 4130–4136 DOI: 10.1021/acs.nanolett.7b00904
Double Dirac point semimetal in 2D material: Ta2Se3. Yandong Ma, Yu Jing and Thomas Heine. 2D Materials, Volume 4, Number 2 (2017).
---
SINC produces scientific news for the European project SCOPE, coordinated by FECYT and funded by the European Union through Horizon 2020, its funding program. The SCOPE mission is to communicate visionary research results of partnering projects in the framework of the Graphene Flagship and the Human Brain Project, as well as to enhance the FET Flagships partnering environment in the European Union.
This is an edited version of a piece produced by SINC which was first published on September 30 2017 under a Creative Commons Licence.
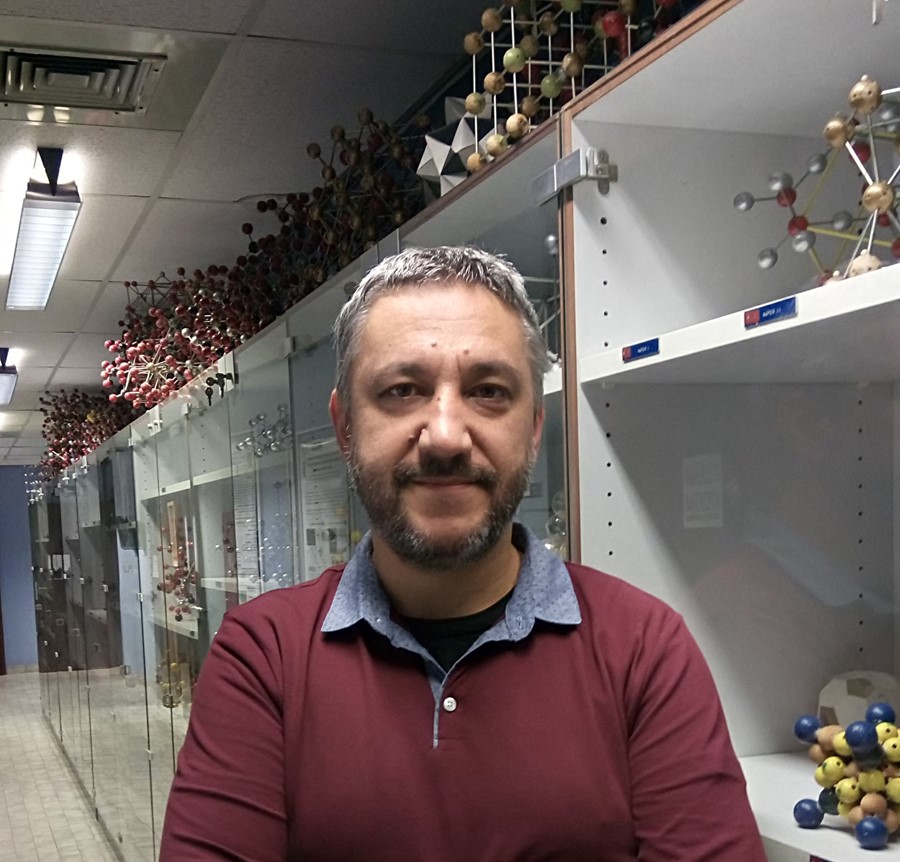
Lucian Covaci of the University of Antwerp