Graphene brings quantum effects to electronic circuits
Two-dimensional materials bring quantum-mechanical effects into the macroscopic world, creating the potential for electronic devices which dissipate very little energy.
Sense and superfluidity
At the atomic and molecular scales, the world can be a very strange place, with everyday notions of temperature, energy and physical coherence thrown into disarray. With reality at the quantum level we must talk of statistical likelihood and probability rather than simple billiard ball cause and effect.
Take the concept of superfluidity, an ultra-cold state in which matter acts as a fluid with zero viscosity. You can think of superfluidity as a generalised thermodynamic analogue of the more commonly understood electrical superconductivity, whereby electrons move through materials without resistance and energy loss.
Bose-Einstein condensation in graphene
Superfluidity was first discovered in liquid helium, at temperatures of just a few degrees above absolute zero, but the phenomenon is evident at scales ranging from the atomic to the cosmic. It is related to the state of matter known as a Bose-Einstein condensate, in which a large fraction of the particles in bulk matter occupy the lowest quantum energy state. The particles, which at higher temperatures move around in a random, haphazard fashion, can in this way behave as a coherent or at least quasi-coherent whole, thus bringing quantum-mechanical effects into macroscopic visibility.
Fascinating if somewhat esoteric physics it may be, but there is a practical side to superfluidity and Bose-Einstein condensation. For one thing it has implications for the behaviour of electronic devices, albeit specialist ones operating at ultra-low temperatures. To this end a group of researchers associated with Europe’s Graphene Flagship have investigated the properties of electrons moving in two-dimensional structures formed from graphene and gallium arsenide.
Graphene and Coulomb drag
Graphene is crystalline carbon arranged in transparent, single atom-thick layers, with the carbon atoms set in a honeycomb-like lattice. The best known of the hundreds of two-dimensional materials discovered to date, graphene has a number of unique electrical, mechanical and other properties that give it huge potential for applications ranging from electronics to super-strong structures.
Focusing on measurements of Coulomb drag – the frictional coupling between electric currents in spatially separated conductors – researchers led by Marco Polini of the Nanoscience Institute of the National Research Council and Scuola Normale Superiore in Pisa, Italy, Vittorio Pellegrini, at the Graphene Labs of the Italian Institute of Technology in Genova, and Andrea Ferrari of the Cambridge Graphene Centre, have found that the drag resistivity increases markedly at temperatures of less than around 5 Kelvin (-268.15 Celsius). This is an unexpected result, departing as it does from the usual temperature dependence displayed in weakly-correlated Fermi liquids: a theoretical model which describes the behaviour of most electrically conductive materials at ultra-low temperatures.
Electron flow in compound 2D structures
In a paper published recently in the journal Nature Communications, the first author of which is Andrea Gamucci, the researchers report on a new class of compound electronic structures in which single or bi-layer graphene is set in close proximity to a quantum well made from gallium arsenide.
A quantum well, formed from a semiconductor with discrete energy values, confines charged particle motion to a two-dimensional plane. Combining graphene with a quantum well results in a heterostructure formed from two different two-dimensional materials, and such a compound assembly may be used to investigate the interaction of electrons and electron holes. A hole is formed when an electron is excited into a higher energy state, leaving in its wake a quasi-particle which behaves as if it were a ‘missing’ electron, or an electron with positive rather than negative charge. Note that electron holes are not the same thing as the physically real anti-particles known as positrons.
Superfluidity in 2D heterostructures
In the case of the graphene-GaAs heterostructures reported in the Nature Communications paper, the Coulomb drag measurements are consistent with strong interactions between the material layers, with the attractive electrostatic force between electrons and holes in solid-state devices predicted to result in superfluidity and Bose-Einstein condensation. In other words, the strong interaction between material layers leads to quantum effects manifest in large ensembles of electrons and holes confined within micrometre-sized devices.
“We show that such effects may happen when electrons are confined in a thin well made of gallium arsenide, with holes confined in monolayer or bilayer graphene,” says Polini. “Electrons and holes separated by a few tens of nanometres attract each other through one of the strongest forces exhibited in nature – the electrical force. At sufficiently low temperatures, our experiments reveal the possible emergence of a superfluid phase, in which opposite currents flow in the two separate two-dimensional systems.” Pellegrini continues: “Such currents flow with minimal dissipation, and may make possible a number of coherent electronic devices which dissipate little energy.” Ferrari adds: “This is an another example of cutting edge results enabled by the deterministic assembly of graphene and other two-dimensional structures, which is precisely the overall target of the Graphene Flagship.”
Graphene in ultra-low temperature electronics
Superfluidity and Bose-Einstein condensation are ultra-low temperature phenomena, so the effects described here in graphene-gallium arsenide heterostructures will not apply to everyday electronic devices. Still, there are many applications which require the use of cryogenically-cooled electronics, and these could exploit anomalous low-temperature Coulomb drag in bulk two-dimensional materials.
Examples of such applications include high-performance and quantum computing, spectroscopy, magnetic and infrared sensing, and analogue-to-digital conversion. The discovery of the Graphene Flagship researchers outlined here could benefit these technology areas and more.
Photo: Andrea Freccioni, Scuola Normale Superiore, 2015
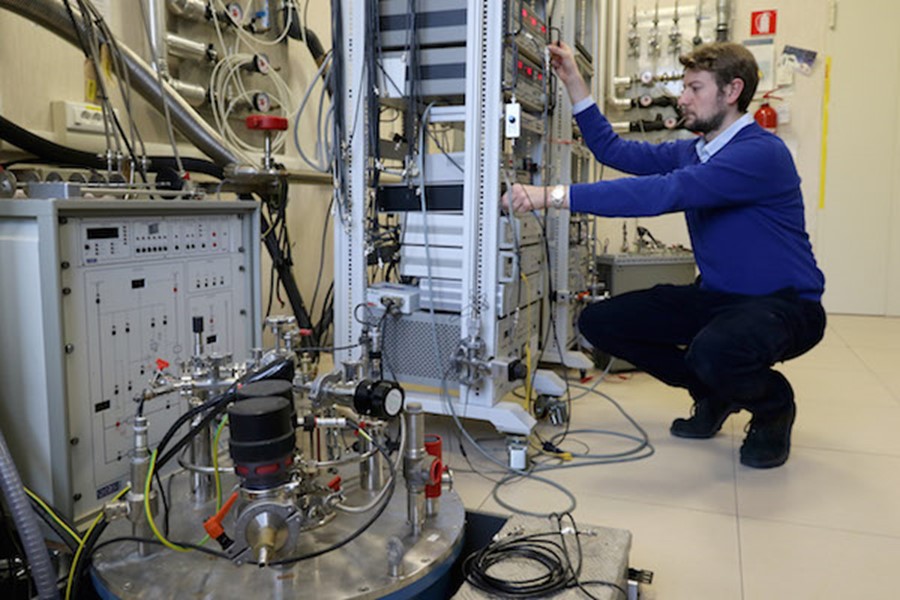